Temperature and salinity in the Fram Strait
Last updated 28 February 2023
The West Spitsbergen Current (WSC) is the only current transporting warm (>3°C) Atlantic water into the Arctic Ocean. Core temperatures are rising and monitoring is needed to predict changes in the Arctic climate system. Heat from the WSC makes Eastern Fram Strait the northernmost perennially ice free sea area in the world and is the dominant factor controlling air temperature over Svalbard.
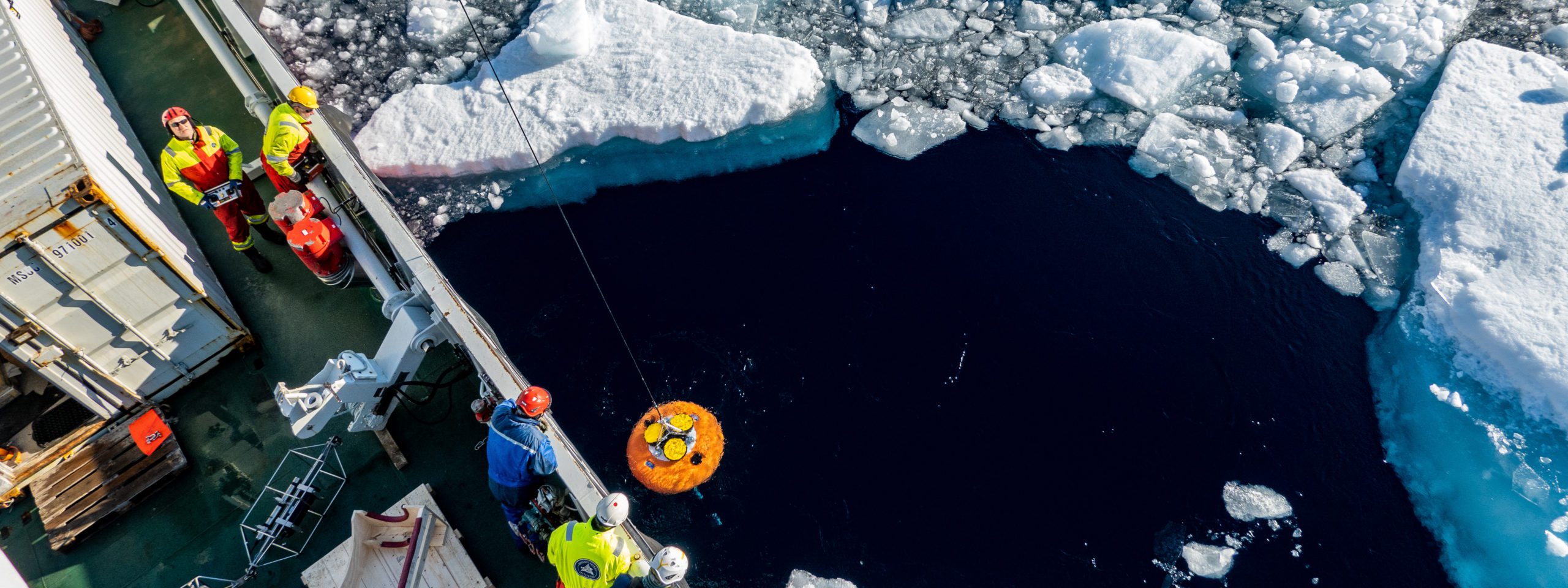
What is being monitored?
Temperature and salinity in the Fram Strait
The core temperature of the WSC (West Spitsbergen Current) is increasing. A similar warming trend is found in year-round measurements from hydrographic moorings in Fram Strait. The change is strongly linked to rising temperatures in the sub-polar north Atlantic. During the recent positive phase of the NAO transport of warm subtropical Atlantic water into Norwegian Atlantic Current has been enhanced. Air temperature over the Nordic Seas and the rate of advection may also affect the temperature of the WSC in Fram Strait.
(Cite these data: Norwegian Polar Institute (2022). Annual maximum temperature in the West Spitsbergen Current. Environmental monitoring of Svalbard and Jan Mayen (MOSJ). URL: https://mosj.no/en/climate/ocean/temperature-salinity-fram-strait.html)
Temperature and salinity in the WSC (West Spitsbergen Current) imperfectly co-vary, with peaks separated by up to 1 year. Comparing the core temperature and salinity of the WSC reveals that warmer profiles are not sufficiently more saline so as to maintain a constant density. The present, warmer WSC is therefore also less dense. Profiles from 1989 and 1993 have especially low salinities in relation to their temperature. These profiles were probably affected by the 1980s Great Salinity Anomaly, which crossed the Sørkapp section south of Svalbard in 1988 and would have reached Fram Strait in 1989.
(Cite these data: Norwegian Polar Institute (2022). Salinity in the core of the West-Spitsbergen current. Environmental monitoring of Svalbard and Jan Mayen (MOSJ). URL: https://mosj.no/en/climate/ocean/temperature-salinity-fram-strait.html)
Details on these data
Last updated | 28 February 2023 |
Update interval | Yearly for yearly maximum temperatures, every 10 years for decadal averages |
Next update | March 2024 |
Executive organization | Norwegian Polar Institute |
Contact persons | Paul Dodd |
Method
The maximum temperature and maximum salinity of the West Spitsbergen Current (WSC) are monitored using hydrographic profiles collected from research vessels in late summer.
The early part (1926-1996) of this indicator is made using hydrographic profiles that were collected by various institutes for different reasons. Early profile positions and collection dates therefore vary from year to year. However, all the profiles used in this indicator fall within a 22-kilometer radius and were collected between 15 August and 30 September.
Strong zonal temperature and salinity gradients at the edges of the WSC mean that profiles need to penetrate the core of the current in order to provide a representative estimate of its temperature and salinity. The WSC follows f/H contours along the shelf break, profiles within 0.25° longitude of the shelf break (as defined by the 500 m isobath) usually penetrate the core of the WSC. Profiles more than 0.25° from the shelf break do not sample the core of the WSC and are not considered in this indicator.
The latitude of profiles is less critical that the longitude. However, the WSC loses heat to the atmosphere resulting in downstream cooling of approximately 0.25 °C per 100 km in the region of Fram Strait (Cokelet et al., 2008). This indicator is calculated using hydrographic profiles from the narrowest range that allows a good temporal coverage: a 44 km wide band between 78° 48’ N and 79° 12’ N. As hydrographic sections are concentrated around 79°N, the band would need to be greatly extended either to the north or south to include significantly more profiles.
From the perspective of this indicator, the latitude of profiles is less critical that the longitude. However, the WSC does lose heat to the atmosphere as it flows northwards, resulting in downstream cooling of approximately 0.25 °C per 100 km in the region of Fram Strait. This indicator is calculated using hydrographic profiles within a 44 km wide band between 78° 48’ N and 79° 12’ N.
Measurements from moored instruments show that WSC has a strong seasonal temperate cycle, with maxima in August/September. Peak-to-trough temperature variations of 1.6 °C occur at the surface above the core and account for > 50% of total variance in that location. The amplitude of the seasonal cycle decreases with depth, falling to 0.5 °C (peak-to-tough) by 200 m where it accounts for < 30% of total variance. It is critical that measurements from different seasons are evaluated separately. The majority of hydrographic surveys in Fram Strait are carried out in August or September, but a significant minority are collected earlier in the year.
This indicator considers profiles collected between 15 August and 30 September (the 6-week period which contains the largest number of hydrographic profiles though the WSC core) from 78° 48’ N to 79° 12’ N and within 0.25° longitude of the shelf break. In years when several qualifying profiles are available, the profile closest to the 500 m isobath is selected. Where multiple profiles were collected at the same distance from the 500 m isobath in the same year, the profile collected closest to 1st September is selected.
Further details about this indicator can be found in NPIs short report 48
http://hdl.handle.net/11250/2500141
Quality
The quality of this indicator will improve if the time and position at which profiles are collected every year can be made more consistent.
Status and trend
The 10-year running mean of (annually interpolated) mean temperatures between 20 and 200 m in the core of the WSC increased from 4.4 °C in 1963 to 5.8 °C in 2016; an increase of 1.4°C in 52 years, or 0.27 °C per decade. No trend is estimated for the period before 1963 as the sparse observations collected before 1963 do not resolve the inter-annual variability that is seen in the later part of the time series.
While there is a clear warming trend, a number of temperature anomalies also occur in the time series. The most pronounced occurred in 2006, when the highest mean core temperature was observed (6.6 °C). Temperatures remained high from 2002-2007 so that the 2006 maximum can be considered part of a 5-year anomalously warm period.
Another warm anomaly occurred in 1984. There is no evidence of a warm period surrounding the 1984 anomaly as there was around the 2006 anomaly, but this may be partly due to a lack of observations in 1985 and 1986. Curiously both the 1984 and 2006 warm anomalies were followed by pronounced local temperature minima 3-4 years later, but with only two cases, this cannot be considered a significant pattern.
Current (2015, 2016), core temperatures are very high and the 4 °C isotherm is currently displaced downwards more than 100 m relative to the 2008-2013 mean depth, as it was during the 2002-2007 warm period surrounding the 2006 warm anomaly.
The temperature profile collected in 1998 is anomalously cool and produces a low spike in the time series. The 1998 profile shows extensive interleaving with a cooler, fresher watermass in the core of the WSC, which is probably not uncommon as there is little density gradient to prevent it. Similar, but less extensive inter-leaving can be seen in the 1987, 1993, 2004 and 2008 profiles. The interleaving seen in the 1998 and other profiles, facilitates the isopyncal mixing though which the WSC loses around half of its heat content.
The mean core salinity varies more smoothly than the mean core temperature. While there was an extreme peak in core temperature in 2006, the core salinity in 2006 was highly elevated for a 3-year period around the temperature peak (2005-2007). The same pattern occurred before the 1984 temperature peak; in both cases elevated salinities were observed in the year before the temperature peak occurred.
Comparing the mean core temperature and salinity of the WSC in each year that observations are available reveals that warmer profiles are not sufficiently more saline so as to maintain a constant density. The present, warmer WSC is therefore also less dense; in 2006 the core was more than 200 gm3 less dense than it was at some times during the late 1970s and early 1980s.
The 1989 and 1993 profiles are some of the least dense recorded, but are not especially warm. The low density is due to a low salinity anomaly which affected the full depth of the water column below the fresh surface layer. These profiles seem to have been influenced by the Great Salinity Anomaly of the 1980s, which crossed the Sørkapp section south of Svalbard in 1988 and would have reached Fram Strait in 1989. These 1989 and 1993 profiles may constitute the furthest downstream observation of the 1980’s Great Salinity Anomaly.
Causal factors
The warming trend detected in the WSC is consistent with observations collected by an array of hydrographic moorings deployed across Fram Strait from 1997 to present (Beszczynska-Möller et al., 2012), but the rates of warming determined by the two approaches are very different: 0.3°C per decade (this study) and 0.6 °C per decade (Beszczynska-Möller et al., 2012). However, it is inappropriate to make quantitative comparisons, as the results are highly dependent on the region and depth range used. Moreover, this indicator captures only the late summer situation while Beszczynska-Möller et al. (2012) describe annual mean temperatures. However, both studies detect the most pronounced anomalies in the time series: the warm/saline anomaly of 2005-2007 and the cold/fresh anomaly of 1998.
Both the long term warming trend and local temperature anomalies observed in the WSC can been linked to changes in the sub polar north Atlantic. Polyakov et al. (2008) find that the upper 2000 m of the North Atlantic warmed by 0.03 °C per decade from 1920 to 2000. Polyakov et al.’s rate of warming is only 1/10th of the rate estimated between 20 and 200 m in the WSC, but is averaged over the top 2000 m. Much of the warming that Polyakov et al. (2008) report is likely to have occurred in the upper layers and the mean temperate change within those upper layers was likely much larger.
Hátún et al. (2005) identify upper ocean temperature and salinity maxima in the sub-polar North Ataltnic in 1997-8 and 2003. Assuming a 2-3 year lag (eg: Polykov et al, 2005) the 2003 anomaly corresponds with the 2006 anomaly observed in Fram Strait. This indicator did not detect a warm anomaly in the WSC corresponding with the 1997-8 anomaly in the sub-polar gyre, but Beszczynska-Möller et al. (2012) observed a warm anomaly in western Fram Strait in 2000 which was presumably conveyed via the WSC.
As the WSC is an extension of the North Atlantic Current, it has been suggested that properties might correlate with the North Atlantic Oscillation index (eg: Polyakov et al., 2008), which entered a positive phase in the mid 1990s after a long negative phase beginning in the early 1960s. During positive phases of the NAO there is an enhanced circulation in the entire meridional overturning circulation, including the northward transport of warm subtropical Atlantic water in the north Atlantic Current.
Holiday et al. (2008) proposed the positive NAO-state as a factor contributing to warming of the Norwegian Atlantic Current from 1995-present, but it is probably not the only factor. Air temperature over the Nordic Seas, which modulates cooling to the atmosphere (eg: Furevik et al., 2001) and the rate of advection (eg: Karcher et al., 2003) have also been proposed as significant influences.
The sparse observational record in Fram Strait is still rather short to fully investigate the causes of inter-decal variability in the properties of the WSC, and that question is beyond the scope of this work, however paleo-oceanographic proxies show that modern temperatures in the WSC are unprecedented over the last 2000 years (Spielhagen et al. 2011).
Consequences
On short time scales, the core temperature of the WSC in Fram Strait is likely to be a good predictor of the local climate and sea ice extent around Svalbard, as local direct heat loss to the atmosphere and strong tidal mixing over shallow bathymetry release heat from the WSC.
Heat supplied by the WSC makes Eastern Fram Strait the northernmost perennially ice free sea area in the world (Haugan, 1999). Piechura and Walczowski (2006) find a strong correlation between the mean summer temperature of the WSC and the ice free area around Svalbard the following winter. The correlation with summer ice free areas is weaker, as solar radiation, wind stress and atmospheric heating also affect the sea ice extent in summer, while in winter oceanic heat input is the only significant factor. There is a higher correlation between local ice cover and the temperature of the northern recirculating branch of the WSC as this branch passes over the shallow Yermak Plateau where warm water has the greatest chance of interacting with sea ice. The Arctic Ocean branch subducts below a polar surface water layer and releases most of its heat further into the Arctic Ocean (Cokelet, et al., 2008). Many processes contribute to the disproportional warming of the Arctic Ocean often called “Arctic Amplification”, but the phenomenon mostly results from the reduction of sea ice extent due to oceanic heat input, which exposes large and dark open water areas where the ocean can absorb more solar radiation and warm further (Overland et al, 2016) a process which is strongly driven by heat supplied by the WSC.
The temperature of Atlantic Water in the WSC is also the dominant factor controlling air temperature over Svalbard (eg: Beuchel et al. 2006). Walczowski and Piechura (2011) find that variations in the temperature of the WSC explain 92 % of the variability in annual mean air temperatures measured at the Hornsund Station.
About the monitoring
The first hydrographic survey in Eastern Fram strait was conducted in 1910, but the first profile that qualifies to be included in the indicator was collected in 1926. Although there were a number of hydrographic surveys in the intervening years, the second qualifying profile was not collected until 1962. Further qualifying profiles have been collected fairly regularly since, excepting a 9-year gap from 1964 until 1973. Since 1997, qualifying profiles have been collected annually as part of the Norwegian Polar Institute’s Arctic Ocean Outflow Observatory in Fram Strait. The first 47 years of the time series are somewhat less illuminating than the more recent part as inter-annual variability is not resolved. Nevertheless, the early part of the time series provides a baseline, which is a relevant indicator for climate change on a centaury scale.
The indicator only describes the late summer situation as there are insufficient observations to build a time series at any other time of year. Augmenting the monitoring program to provide winter observations would be worthwhile, as winter is the season when the link between local sea ice extent and ocean heat input is strongest (Piechura and Walczowski, 2006) and late summer observations of the WSC may not accurately describe the winter situation, when different processes may dominate.
Places and areas
A number of stations. The number of instrumental observations of temperature in the Fram Strait is not uniform in time and space. Most of the observations were carried out in the past four decades in the summer season. Since the end of the 1980s, the observations in the 79°N transect in the Fram Strait have been performed annually on a regular basis. Therefore, for monitoring purposes we use only the observations in summertime (July–September) between latitudes 78°30N and 79°30N.
Relations to other monitoring
Monitoring programme
International environmental agreements
- None
Voluntary international cooperation
Related monitoring
- None
Further reading
Links
Publications
- Beszczynska-Møller, A., Fahrbach, E., Schauer, U., & Hansen, E. (2012). Variability in Atlantic water temperature and transport at the entrance to the Arctic Ocean, 1997–2010. ICES Journal of Marine Science 69: 852–863. https://doi.org/10.1093/icesjms/fss056.
- Beuchel, F., Gulliksen, B., & Carroll, M. L. (2006). Long-term patterns of rocky bottom macrobenthic community structure in an Arctic fjord (Kongsfjorden, Svalbard) in relation to climate variability (1980–2003). Journal of Marine Systems, 63(1-2), 35-48. https://doi.org/10.1016/j.jmarsys.2006.05.002.
- Cokelet, E.D., Tervalon, N., & Bellingham, J.G. (2008). Hydrography of the West spitsbergen current, svalbard branch: autumn 2001. Journal of Geophysical Research: Oceans, 113(C1). https://doi.org/10.1029/2007JC004150.
- Furevik, T. (2001). Annual and interannual variability of Atlantic Water temperatures in the Norwegian and Barents Seas: 1980–1996. Deep Sea Research Part I: Oceanographic Research Papers, 48(2), 383-404. https://doi.org/10.1016/S0967-0637(00)00050-9.
- Haugan, P.M. (1999). Structure and heat content of the West Spitsbergen Current. Polar Research, 18(2), 183-188. https://doi.org/10.3402/polar.v18i2.6572.
- Hátún, H., Sandø, A. B., Drange, H., Hansen, B., & Valdimarsson, H. (2005). Influence of the Atlantic subpolar gyre on the thermohaline circulation. Science, 309(5742), 1841-1844. https://doi.org/10.1126/science.1114777.
- Karcher, M. J., Gerdes, R., Kauker, F., & Köberle, C. (2003). Arctic warming: Evolution and spreading of the 1990s warm event in the Nordic seas and the Arctic Ocean. Journal of Geophysical Research: Oceans, 108(C2). https://doi.org/10.1029/2001JC001265.
- Overland, J.E. (2016). Is the melting Arctic changing midlatitude weather?. Physics Today, 69(3), 38-43. https://doi.org/10.1063/PT.3.3107.
- Piechura, J., & Walczowski, W. (2009). Warming of the West Spitsbergen Current and sea ice north of Svalbard. Oceanologia, 51(2), 147-164. http://dx.doi.org/10.5697/oc.51-2.147.
- Polyakov, I. V., Beszczynska, A., Carmack, E. C., Dmitrenko, I. A., Fahrbach, E., Frolov, I. E., … & Walsh, J. E. (2005). One more step toward a warmer Arctic. Geophysical Research Letters, 32(17). https://doi.org/10.1029/2005GL023740.
- Polyakov, I. V., Alexeev, V. A., Bhatt, U. S., Polyakova, E. I., & Zhang, X. (2010). North Atlantic warming: patterns of long-term trend and multidecadal variability. Climate Dynamics, 34, 439-457. https://doi.org/10.1007/s00382-008-0522-3.
- Spielhagen, R. F., Werner, K., Sørensen, S. A., Zamelczyk, K., Kandiano, E., Budeus, G., … & Hald, M. (2011). Enhanced modern heat transfer to the Arctic by warm Atlantic water. Science, 331(6016), 450-453. https://doi.org/10.1126/science.1197397.
- Walczowski, W., & Piechura, J. (2011). Influence of the West Spitsbergen Current on the local climate. International journal of climatology, 31(7), 1088-1093. https://doi.org/10.1002/joc.2338.